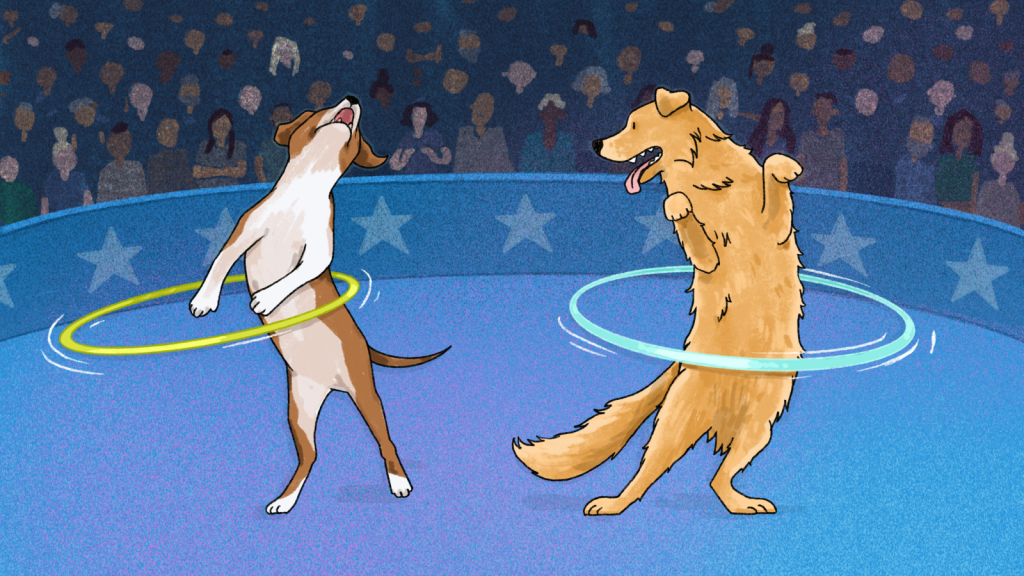
In 1995, Alexander Grohsjean cut out a story from the local German newspaper Saarbrücker Zeitung. He was 16 years old and mystified by what he read.
“I brought it to school because I wanted to find a teacher who could explain it to me, but I failed,” he says.
The story was about the top quark—the heaviest known fundamental particle—which had just been discovered by scientists at the US Department of Energy’s Fermi National Accelerator Laboratory. Even though he didn’t fully understand it, the story intrigued Grohsjean.
“That was really what triggered my interest,” he says.
Today, Grohsjean is a particle physicist working on CMS, one of the main experiments collecting data from the Large Hadron Collider at CERN. His career in physics led him to Fermilab, then Paris, and finally back to Germany where he is now a researcher at the University of Hamburg. It also led him back to the top quark, which he hopes can be a window to discover even more particles.
“The top quark is special,” he says. “It is the heaviest particle, which gives it an unusual connection to the Higgs boson.”
Recently, Grohsjean and his colleagues published an analysis that looks at collisions that generate two top quarks simultaneously and found something unexpected: a large excess of events that could be indicative of a new particle. But what these findings mean has caused a stir in the community that is still far from resolution.
A very quirky quark
Physicist Christian Schwanenberger has been studying the top quark since 2004.
“I arrived a Fermilab nine years after the discovery,” says Schwanenberger, a scientist at DESY and professor at the University of Hamburg. “The top quark was new, and we needed to figure out how to measure its properties. It was a really creative time.”
At Fermilab, Schwanenberger and Grohsjean both wanted to know if twin top quarks born from a single collision experienced something called spin correlation. Spin is a fundamental property, like mass or charge, and describes a particle’s intrinsic angular momentum. Spin correlation is the relationship between two particles’ spins.
“When we produce a pair of top quarks, we can use our theories to predict how many pairs are spinning in the same direction versus how many pairs are spinning in opposite directions. And this property tells us something about how the top quarks are produced,” Grohsjean says.
In 2011, Schwanenberger, Grohsjean and their collaborators on the DZero experiment at Fermilab’s Tevatron accelerator found the first evidence of spin correlation in top quarks, proving that this fundamental property was indeed measurable in particle collisions. When the Large Hadron Collider started collecting data in the 2010s, Schwanenberger and Grohsjean continued this research on the ATLAS experiment, where they confirmed the results from DZero with much higher statistical certainty. They then switched to CMS, at which point they were ready to use spin correlation to push into new frontiers.
“Spin correlation is a very powerful tool to look for new physics,” Grohsjean says.
Scientists can precisely predict the ratio of top quark pairs with parallel spins (both spinning in the same direction) versus those with antiparallel spins (spinning in opposite directions) based on how top quarks are produced. For instance, when top quark pairs are created through the strong interaction, the Standard Model predicts that about two-thirds of them will have parallel spins. However, if an unknown exotic particle contributes to top quark production, this ratio could deviate from the predictions.
In 2016, Grohsjean and Schwanenberger started to explore spin correlation to look for heavier cousins of the Higgs boson. The Higgs boson was discovered at CERN in 2012 and helps describe why some particles are massive while others are not. The Standard Model only predicts one Higgs boson, but some theorists think that there could be more.
“We were really looking for new, beyond-the-Standard-Model particles; that’s why we did this search,” Grohsjean says. “If they are heavy enough, these massive Higgs bosons would first show up in top quark production.”
In 2019, Schwanenberger, Grohsjean and their colleagues on CMS found something unexpected that looked like the signature of a new particle: a small excess of top quark pairs with antiparallel spins right at the lowest possible energy for top quark pair production.
“We were really excited,” Schwanenberger says. “But it was so small that it could have just been a statistical fluctuation.”
What they needed was much more data and a more sophisticated analysis.
“For this new analysis, we used all the data collected between 2016 and 2018, which had also been recalibrated and reprocessed by CMS scientists to lower the systematic uncertainties,” Grohsjean says.
When the scientists finally unveiled the data in October 2024, they had a surprise. The small excess they had seen in 2019 was now massive and well beyond the threshold for discovery.
“It’s like winning the lottery for new physics effects,” Schwanenberger says.
The team was now sure that they were seeing something new, and they had a few ideas about what it could be. But before claiming a discovery, they wanted to find out what the theorists had to say.
Don’t call it toponium
While the CMS scientists were exploring the top quark’s secrets with a collider, members of the theoretical physics community were debating a key tenet of the top quark’s personality: whether it could momentarily bond with other top quarks.
When the LHC started up in 2008, theorist Fabio Maltoni read a paper about a theoretical top-anti-top bound state called toponium. “I remember thinking, toponium at the LHC is a fun thing, but it will never be seen,” says Maltoni, a professor at Università di Bologna and Université Catholique de Louvain.
As a PhD student, Maltoni studied the bound states of quarks with a focus on heavy quarks like the bottom and charm. Quarks are social particles and will always clump up and form hadrons like protons and neutrons (which are made from light quarks) or mesons that consist of a quark anti-quark pair. The one exception was the most unstable quark, the top quark.
“Even when I was doing my PhD, people were talking about toponium, but we all knew that the top quark decays so quickly that it cannot form a bound state,” he says.
Nonetheless, the idea wedged itself into Maltoni’s head, and as more people started to talk about toponium in both the experimental and theoretical physics communities, Maltoni became excited again about top quark bound states.
The theory was that if two top quarks were produced simultaneously and moved very slowly, they might see each other, exchange a gluon and start to orbit.
“It’s the Romeo and Juliet of particle physics,” Maltoni says. “As soon as they see each other by exchanging a gluon, one of them disappears.”
In January 2024, Maltoni and his colleagues proposed that if top quarks could dance with each other—even just for half a twirl—the LHC experiments might be able to detect it. It would appear as an excess of top quark pairs materializing at the lowest possible energy with very specific quantum spin states. This happened to be exactly the region Schwanenberger and Grohsjean had been studying since 2016.
“When we started the analysis, we did not think about toponium,” Schwanenberger says. “If you look at the textbooks, they say that there is no toponium.”
But as other analysis groups also started to see excesses in the same region as Schwanenberger and Grohsjean, the toponium debate gained momentum, and experimentalists and theorists came together to confer.
“I talked to one theorist who said he would never call it toponium,” Schwanenberger says. “But then his collaborator was putting all their results into a folder called ‘toponium.’ Even people within the same working group have different opinions.”
There is no question that Grohsjean, Schwanenberger and their colleagues on CMS have seen something unexpected.
“This enhancement looks like toponium, walks like toponium and quacks like toponium,” Maltoni says.
However, scientists still cannot rule out other possible explanations for the excess. “Experimentally, the observed excess could be from a top quark quasi-bound state or from a new heavy Higgs boson,” Grohsjean says. “But the location of the excess is curious, since it is right where toponium should be. It would be really surprising to see a new particle in the exact same location and with exactly the same quantum properties.”
For Schwanenberger, while the semantics are still important, toponium by any other name would still be just as sweet.
“If I go to bed and start thinking about toponium, I cannot sleep,” Schwanenberger says. “It’s probably not a Nobel Prize winning discovery, but it’s still something exciting. As a scientist we want to do something that will survive our careers, even if it’s just a small update to the physics textbooks.”